Challenges in the development of quantum technologies
Quantum technologies over the past 10 years have begun to move out of university laboratories worldwide and be tested in real world applications [5] [6] [7] [A]. To enable this, many technological and scientific challenges have had to be overcome.
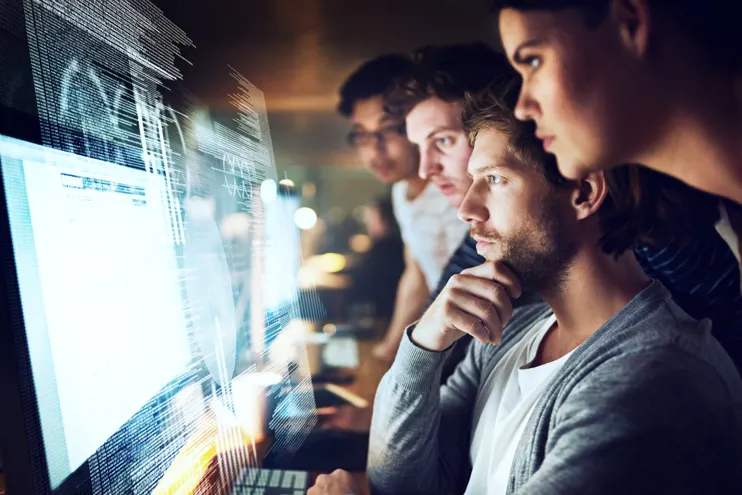
Quantum technologies, due to the way they harness principles of quantum physics, are fundamentally different from classical technologies.
These differences, along with the current low state of readiness for quantum technologies, mean that there is much work to do to integrate them effectively into the multitude of desired applications and create exportable products that will result in economic benefits to the UK.
The key to addressing this challenge and enabling a rapid route to market is to ensure that the existing systems engineering methodologies used for classical technologies can be extended, or new methodologies created, to handle the unique properties found in the quantum case.
Quantum navigation - application example
Many existing navigation systems rely on the use of global navigation satellite systems (GNSS), which use signals from satellites. However, while GNSS navigation can be very accurate, it is not always accessible. Obstacles, such as buildings and natural features, such as canyons, can block the satellite signals.
In addition to this, GNSS systems are also susceptible to jamming, imitation, or denial, resulting in negative impacts to platforms utilising GNSS in their navigation systems. It has been estimated that a single day of GNSS service denial would result in a cost of £1 billion to the UK [8].
When GNSS systems can’t be used, a number of satellite-free navigation methods can be used, however such systems are limited in their effectiveness. For example, when using inertial navigation, the process of navigating via the use of measurements of rotations and accelerations, the sensors currently used drift over time, resulting in reduced accuracy unless they are regularly calibrated with GNSS.
Quantum sensors have the potential to overcome these limitations, due to their low drift and high accuracy. Quantum sensors of interest in navigation include quantum accelerometers, quantum gyroscopes, quantum clocks, quantum magnetometers, and quantum gravity sensors. By incorporating the new generation of quantum sensors into navigation systems, improved performance in the absence of GNSS is expected, for example, when GNSS is denied through jamming or spoofing.
However, before the benefits of quantum sensors can be realised in a navigation system, there are several challenges that need to be overcome in the sensors themselves, including improving their robustness and reducing their size, weight, power consumption, and cost.
In addition to the challenges in developing the sensors themselves, there are a number of challenges which need to be overcome in the integration of the sensors [B] [C] [D], including the data fusion of quantum sensors with classical ones.
Quantum enhanced radar - application example
Radar surveillance is used in a number of applications and locations, including urban environments, for the radar surveillance of small objects in low- to medium-altitude airspace. The surveillance of targets includes the detection, tracking and identification of targets in the location or locations of interest. Other applications for radar surveillance include counter-drone surveillance, drone traffic management and agroecology.
However, in urban environments, weak targets (e.g. drones, birds) can be masked by phase noise introduced by the local oscillator used in radar systems, and this can fundamentally limit the radar sensitivity. Advances in Quantum Technologies offer a potential solution to this problem through the integration of quantum clocks as ultra-stable oscillators into radar systems.
It is expected that this will reduce signal noise, enabling radar systems to detect small, slow-moving objects such as drones at longer distances and in more cluttered environments. Further benefits of quantum clocks in radar systems are expected when incorporated into next-generation distributed radar systems, which will allow surveillance with greater coverage and increased ability to detect stealthy objects.
However, before quantum-enhanced distributed radar systems can be realised, there are several challenges which need to be overcome in the clocks themselves, including improving the robustness, and reducing their size, weight, power consumption and cost.
In addition, there are challenges in how best to integrate quantum clocks into distributed radar systems, including, for example, how to distribute the new levels of timing accuracy without reducing its quality.
References
A Boto, E., Holmes, N., Leggett, J. et al. Moving magnetoencephalography towards real-world applications with a wearable system. Nature 555, 657–661 (2018). https://doi.org/10.1038/nature26147
B Cheiney, Pierrick, et al. "Navigation-compatible hybrid quantum accelerometer using a Kalman filter." Physical Review Applied 10.3 (2018): 034030. https://doi.org/10.1103/PhysRevApplied.10.034030
C Wang, Xuezhi, et al. "Improving measurement performance via fusion of classical and quantum accelerometers." The Journal of Navigation 76.1 (2023): 91-102. https://doi.org/10.1017/S0373463322000637
D Tennstedt, Benjamin, et al. "Atom strapdown: Toward integrated quantum inertial navigation systems." NAVIGATION: Journal of the Institute of Navigation 70.4 (2023). https://doi.org/10.33012/navi.604
Thanks for your patience. We’ve upgraded our systems, all part of our big picture plan to deliver a great experience for you.
Your log-in access has been reinstated for our websites and systems but should you encounter any issues, our Member Relations team is here to support you on +44 (0)1438 765678 or via membership@theiet.org.